Published on 17th of October 2023 – Frederik K. Marqversen, Quantum Engineer – Kvantify
A wide variety of hardware platforms are competing to be the first to demonstrate large scalable quantum computing, and part of the Kvantify mission is to help our partners maintain an overview of the ever-evolving strengths and weaknesses of each platform.
In this blog post we will present our perspective on the efforts toward photon-powered quantum computing which has seen several technical breakthroughs in recent years.
Starting from the fundamentals and then diving into our recent article on applications and resource reductions in measurement-based variational quantum eigensolvers, we will explore the advantages of using photonic systems and examine the drawbacks and challenges associated with the technology.
Photon numbers: The quantum packets of light
The first question that we need to answer is: How can information be encoded into something like light? In quantum mechanics, a state of light consists of a collection of individual particles called photons. And so, the most direct way of representing information is in the number of photons that are present.
As an example, suppose you are given some packet of light and can discern that it contains a total of 4 photons. Then, this packet of light we can think of as representing the number 4. Since photons are quantum objects, and as such exhibit quantum effects such as superposition and entanglement, this encoding presents a valid platform for quantum computations.
But why should we care about using photons for quantum computers? Well, there are certain advantages one could discuss. Here I picked two that distinguish it from superconducting quantum computers.
Qumodes: Utilizing the mobility and indistinguishability of photons.
One of the big disadvantages of superconducting quantum computers is that the information is stored on qubits that exist in fixed positions on the superconducting chip. This means that if you want to have two qubits talk to each other, you must first go through a difficult procedure of swapping the qubits around to bring the ones you are interested in close to each other.
In contrast, light moves through space at high speed by itself, making it a flying carrier of information. Importantly, using what are called waveguides, it is possible to make light, and hence the information it contains, go exactly where we want it to go, e.g., close to other light for interaction purposes or simply into our detectors.
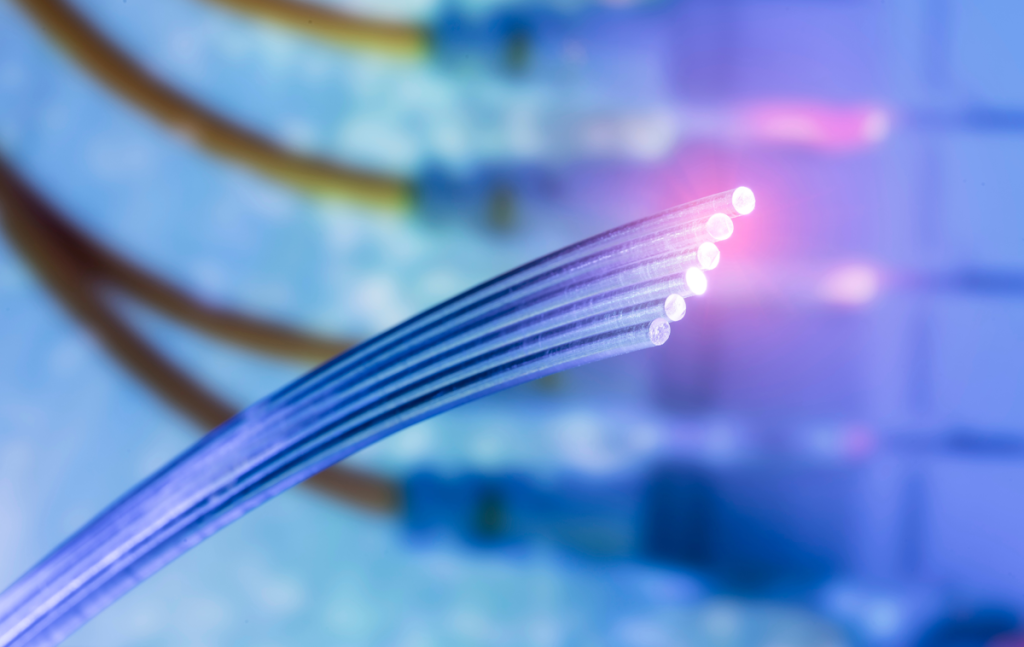
Another important concept to unravel is the indistinguishability of photons. Not all photons are equal. There are properties like energy, spatial location etc., that they can possess that make them distinguishable from one another. However, photons that share these properties are in fact truly and fundamentally identical. This means that photons bunch up in packets that act as individual units. These packets we call qumodes.
All physical quantum hardware is inherently noisy, and a key challenge in building a quantum computer is to come up with strategies to ensure that the system can tolerate such noise. Since a qumode can contain any whole number of photons, qumodes can store much more than just qubit information. This type of abundance of informational space allows for encoding the quantum information in clever ways as to increase fault-tolerance.
Navigating Limitations: The Challenge of Achieving Universal Quantum Computing with Light
One of the important concepts of quantum computing is that of the circuit model. In short, a circuit is a sequence of simple operations called gates, together forming a new and more complicated operation. It turns out that any conceivable operation, no matter how complicated, can be realised as a circuit built from a small finite set of gates. Such a set is called a universal gate set. If a system is to be considered interesting for quantum computing, it should be possible to perform all kinds of operations, and so, it is important that a universal gate set is available.
Unfortunately, it is not entirely smooth sailing for photonics. It turns out that the operations that we are particularly good at executing with light, formally called Gaussian operations, are not sufficient for universal quantum computing. Therefore, without including some difficult and typically lossy operations, the possibilities will be very restricted.
Also, photon counting is hard. The way we detect photons is by measuring their energy. But the energy of a single photon is very small, much smaller than typical thermal noise at room temperature. Therefore, to resolve the energy of a single photon, detectors must be cooled to superconducting temperatures. However, we are actually pretty good at cooling, so this is not the biggest issue. The issue lies in detecting and differentiating large numbers of photons. When a lot of photons are absorbed by the detector, the temperature rises correspondingly which in turn reduces the resolution making it very hard to tell apart say 100 and 101 photons. Therefore, algorithms must be designed with this limitation in mind.
Gaussian Boson Sampling: Charting the Realm of Quantum Computation with Current Resources
So, what is possible with the equipment and methods we have today? If we put together Gaussian operations and photon counting, then this is what we call Gaussian boson sampling (GBS). Even though several such devices already exist, only one or two of these are available to the public for testing, limiting the progress in the field. For that reason, the Innovationsfonden funded project PhotoQ is aiming to build a fully configurable GBS device publicly available through the cloud.
This might seem a bit silly my above comments considered. However, there is reason to believe that GBS is hard to reproduce classically, and therefore might have some computational advantage over classical computing. Also, studies exist that have presented use cases for such devices, spanning from abstract graph problems to molecular simulations. Finding good use cases, however, is a particularly challenging problem due to the restricted class of operations available and the non-perfect photon counting detectors. At Kvantify we are actively working with the PhotoQ project on these particular subjects, hoping to, at some point, show the utility of GBS.
Measurement-Based Quantum Computing: Advancing Photonic Systems Toward Universality
GBS in itself is not the end goal for photonic quantum computing, due to it not being universal. A universal system is capable of so much more. So, what are the prospects of universal quantum computing on photonic systems? Well, one of the more promising ways forward is something called measurement-based quantum computing. The counterintuitive nature of entanglement and quantum measurements makes it possible to act on one part of a quantum system by measuring in an entirely different part of that system. The result of the measurement will depend on the entanglement in the system and the measurement performed.
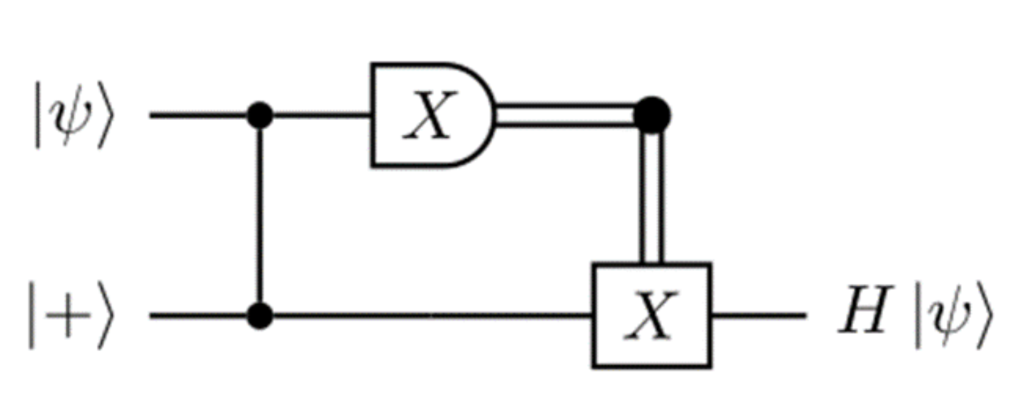
However, by cleverly engineering some specific systems and doing the right measurements complex quantum operations can be enacted. Measurement-based computations are for that reason also a part of the PhotoQ vision and something that we study at Kvantify.
But why is measurement-based quantum computing especially interesting in discussing photonics? There is this particular type of state that can be made in a photonic system called a GKP state. It turns out, that with a source of GKP states, it is possible to do universal quantum computations through measurements using nothing but Gaussian operations (remember these are the ones we already excel at) and importantly also without the need for difficult photon counting.
Here it should be noted, that finding a way of producing good-quality GKP states is an extremely challenging problem and an active area of research. However, I would be surprised if this single although important puzzle piece is not solved sometime in the future. In the meantime, we can prepare by designing and testing promising algorithms for the framework.
For more information on our quantum-ready solutions, please do not hesitate to reach out at contact@kvantify.dk